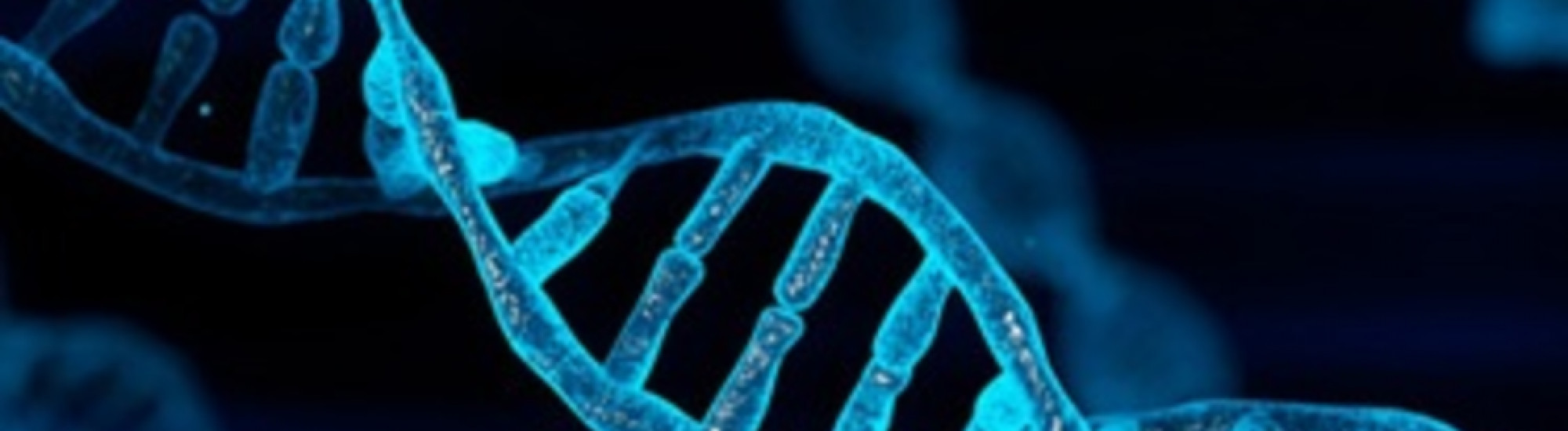
By Dr. Catherine Jomary, Technology Lead ATMPs
Although messenger RNA (mRNA) based therapeutics have been explored for over two decades, none have reached commercialization until the first SARS-CoV-2 mRNA vaccines were developed and launched in less than 12 months by BioNtech/Pfizer and Moderna. This acceleration is due to technological advancements in RNA biology, chemistry, stability, and improved delivery system. Going forward, mRNA technology will not only help the development of other vaccines for infectious diseases, but also address non-viral gene and cell therapy applications for diseases with unmet needs.
The process of producing mRNA from a DNA template is cell-free and scalable but plasmid DNA manufacturing requires microbial fermentation. The full manufacturing process from DNA template to mRNA encompasses 3 main stages summarized below:
- The target DNA sequence, corresponding to the protein of interest blueprint, is introduced into a plasmid (pDNA). This plasmid is then amplified using bacterial fermentation (i.e. E coli), purified and linearized.
- The mRNA is synthetized during an enzymatic reaction called in vitro transcription (IVT). The template - linearized pDNA - containing the target DNA sequence, nucleotides and enzymes are mixed together. The resulting transcribed mRNAs are then purified from the reaction contaminants using chromatography, tangential filtration, and sterile filtration.
- The final step is to encapsulate the mRNAs in lipids, and the final product is purified and concentrated using chromatography and tangential flow filtration (TFF) prior to sterilisation and filling.
The full process presents several manufacturing challenges to be overcome. Part 1 of this review will suggest technical remedial options for the production of pDNA. Part 2 of the series will focus on mRNA synthesis, purification and encapsulation.
Part 1 pDNA Manufacturing Challenges
Since the starting point manufacturing mRNA is pDNA (circular, double-stranded DNA), its design and purity are essential to optimize mRNA products. As such, pDNA is considered as a critical starting raw material for mRNA manufacturing. Plasmid exist in several isoforms, the supercoiled plasmid is the active form (fully intact and wound around itself), while the open circular (one strand is broken and the molecule relaxes) and linear (both strands are broken with free ends) forms are considered impurities. The main pDNA manufacturing process encompasses several cell bioprocessing steps:
- E. coli fermentation - pDNA amplification
- E. coli cell harvest
- Alkaline cell lysis to release pDNA from E. coli
- Clarification, concentration and diafiltration – pDNA purification first steps
- pDNA purification by chromatography, concentration and diafiltration
- pDNA final sterile filtration prior to sterile fill
The three main challenges to manufacture large size pDNAs are (i) high viscosity of plasmid solutions, (ii) high sensitivity to shearing, and (iii) similarity with some impurities (linear and open circular pDNA). They all have an impact on several manufacturing process steps that will be discussed below.
During E. coli harvest, centrifugation (commonly used during the R&D project phase) has been shown to induce DNA shearing. In contrast, microfiltration combined with TFF will avoid this type of damage. These two methods have the advantage to enable linear process scalability. Besides, a wide range of equipment format and fitting that can accommodate different plasmid manufacturing requirements are available off-the-shelf from several suppliers.
Alkaline cell lysis, that releases E. coli cell contents, is performed at a pH closed to the pH that can denature DNA. High viscosity of the lysis mixture can also damage the supercoiled pDNA. Using equipment that can control not only the pH during the addition of alkaline solution, but also the mixing rate of the mixture, can prevent these types of damage.
The resulting viscous lysate mixture is a complex combination of cell debris and genomic E. coli DNA found at the top of the lysate solution. pDNA, E. coli RNA and cells proteins are localized in the underneath layer. Clarification step removes the impurities found at the top of the viscous lysate mixture. Precipitation of E. coli RNA can be achieved by adding, for example, CaCl2 to the lysate mixture. Various methods have been used to improve this clarification step, centrifugation is again not recommended to avoid DNA shearing. Flow filtration using size exclusion and adsorption mechanisms are more appropriate and constitute a robust clarification method. Depth filters have demonstrated excellent performance over a wide range of conditions, making them a viable platform option. Many installation options and simple scaling capability make this approach the most suitable to improve clarification at large scale.
An additional TFF step can be added between clarification and chromatography steps. This step washes out residual impurities through diafiltration, and reduces the volume of impurified pDNA solution prior to chromatography steps.
Chromatographic purification of pDNA can utilize either resins or membranes. Resins are characterized by good selectivity and flexibility, but plasmids are too large to diffuse through the resin beads reducing the resin binding capacity and making this purification method potentially sub-optimal. Alternatively, chromatography membranes binding site capability and flow rates are higher with large pore membranes, making it the most appropriate method to purify large plasmid. Depending on the optimum process develop during R&D, either resins or membranes can potentially be used for pDNA purification.
At the end of the process, pDNA formulation buffer is exchanged by diafiltration. The resulting pDNA solution is then concentrated to the chosen final concentration using TFF. The final sterile filtration can be challenging due to large size of some plasmids and high viscosity of the solution. Thorough process development should be performed, in particular for larger plasmids above 20 kilobase pairs that often encounter sterile filtration yield and capacity issues.
To achieve reproducible and scalable manufacturing of pure pDNA, process development is key to overcome challenges associated with plasmid attributes - high viscosity, high sensitivity to shearing and similarity with impurities. Taking advantage of single-use technology and automation will increase safety, reliability, efficiency, low-cost and flexibility to allow on-demand manufacturing that meet regulatory requirements.